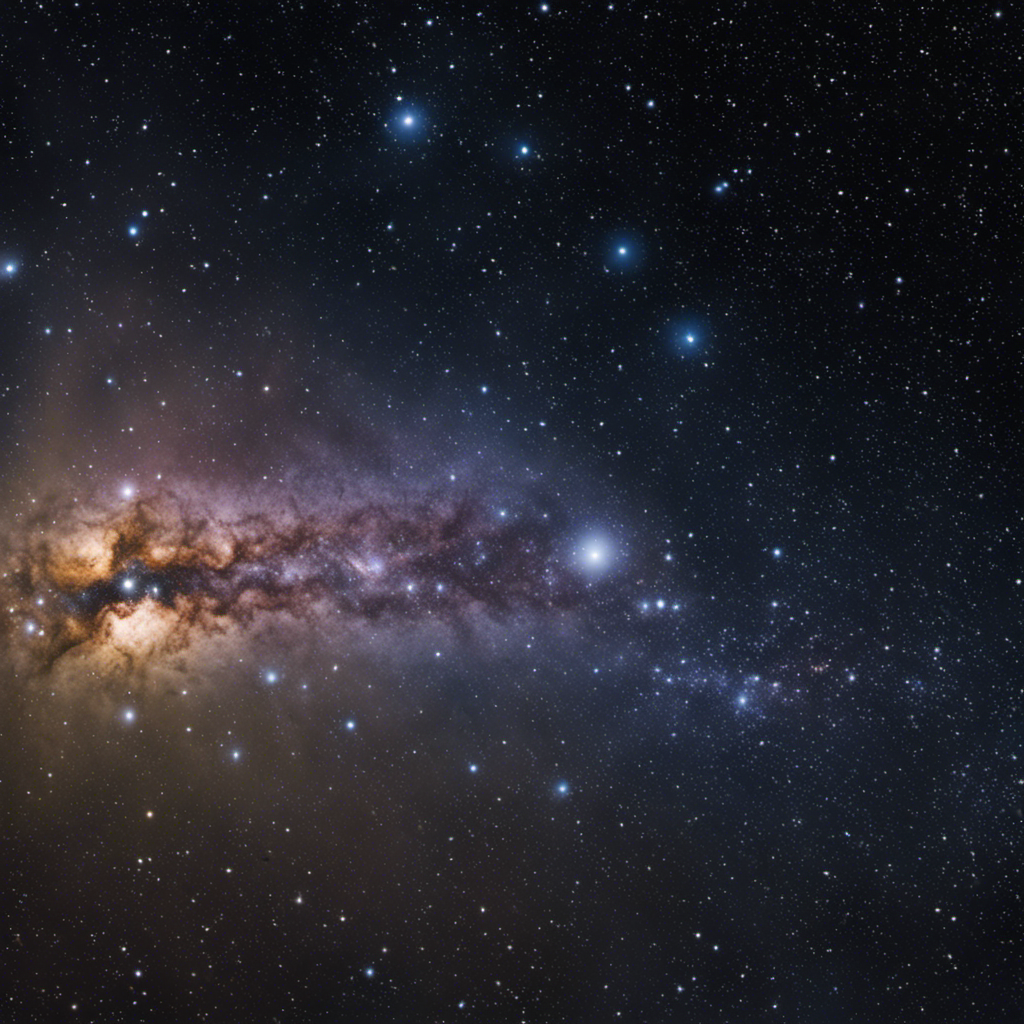
No one was surprised last week when researchers with a massive new dark matter detector called LUX-ZEPLIN (LZ) reported finding nothing. After all, LZ started hunting its quarry, hypothetical weakly interacting massive particles (WIMPs), a few months ago and has collected just a few percent of its expected data. Still, the announcement piqued the interest of physicists. LZ, a U.S. detector, is turning on at the same time as similar detectors in Italy and China. They likely mark the final or nearly final phase in the decadeslong quest to spot these particles, a potential solution to one of physics’ greatest mysteries.
The three-way competition to spot WIMPs will push all the groups to work harder, says Dan Hooper, a theorist at Fermi National Accelerator Laboratory, who was not involved in the work. “The physicist-capitalist in me thinks this is a good thing,” Hooper says.
Dark matter is thought to account for 85% of all matter. Astronomical observations show, for example, that the stars in a typical galaxy swirl so fast that their collective gravity isn’t strong enough to keep them from flying into space. So physicists assume the extra gravity needed to rein in the stars comes from invisible dark matter—presumably consisting of some new fundamental particle.
Since the 1980s, many physicists have favored WIMPs, which would interact with ordinary matter just through gravity and the weak nuclear force. WIMPs would have emerged naturally after the big bang, and enough of them should linger to account for dark matter, provided they are about 100 times as massive as a proton. They would drift unobtrusively through the Galaxy and even our own bodies, but occasionally one ought to crash into an atomic nucleus. So, physicists stalk WIMPs by looking for recoiling nuclei in detectors deep underground, where they’re shielded from radiation that can produce extraneous signal.
For 20 years, scientists have developed ever-bigger detectors consisting of tanks of liquid xenon lined on top and bottom with light-detecting phototubes, which detect the flash of light produced by a recoiling nucleus. Simultaneously, an electric field tugs electrons liberated by the speeding nucleus out of the liquid, producing a second flash. Comparing the sizes and times of the flashes, researchers can distinguish recoiling nuclei from, say, recoiling electrons, which can be generated by gamma rays hitting the detector.
Filled with 7 tons of liquid xenon and hunkering 1480 meters down in the Sanford Underground Research Facility in Lead, South Dakota, LZ is the biggest liq-uid xenon detector yet. In its first 65 days of data taking, it detected 335 recoil events, Hugh Lippincott, a physicist at the University of California (UC), Santa Barbara, and spokesperson for the 287-member LZ team, reported on 7 July in an online seminar. But roughly that many background events are expected from inevitable traces of radioactive radon in the liquid and other sources, Lippincott reported, so there’s no evidence of recoils coming from WIMPs.
That null result puts the strongest limits yet on how strongly WIMPs with masses between about 10 and 10,000 times that of a proton could interact with ordinary matter. LZ’s new limits edge past those published in 2021 by a team using PandaX-4T, a 3.7-ton liquid xenon detector in the China Jinping Underground Laboratory. A third detector, XENONnT, has a 5.9-ton central chamber and has been taking data in Italy’s subterranean Gran Sasso National Laboratory. Initial results are due later this year, says Rafael Lang, a physicist and XENON team member at Purdue University.
Some of the enthusiasm for WIMPs as dark matter candidates has waned in recent years, not only because searches have come up empty so far, but also because the world’s biggest atom smasher, Europe’s Large Hadron Collider, has yet to blast out anything that looks like a WIMP. However, physicists are only now starting to probe the heart of the possible ranges of mass and interaction strength for WIMPs, Lang says. “Half of the [possibilities] that you were excited about a decade or two ago are still alive and well.”
Largely by chance, all three new detectors have come to life at roughly the same time. “Five years ago, you couldn’t have predicted them to all come on within months of each other,” Lippincott says. Having three different experiments running simultaneously provides an advantage should any of them see a signal, says Katherine Freese, a theoretical astro-physicist at the University of Michigan, Ann Arbor. “You always need to reproduce the results with a different experiment.”
The current detectors should run for 3 to 5 years. In case they come up empty, WIMP hunters are already sketching out the ultimate liquid xenon detector, an 80-ton giant. In June, the LZ and XENON teams met to begin planning such a device. “We aim to combine the best aspects of the two [current detectors],” says XENON member Laura Baudis, a physicist at the University of Zürich.
A detector that large would probably mark the end of the line. It would be sensitive enough to begin to detect a “fog” of particles called neutrinos from the Sun and the atmosphere, which it could not distinguish from WIMPs, notes Jonathan Feng, a theorist at UC Irvine. An even bigger liquid xenon detector would make little sense. Still, Feng says, “There’s no good reason to stop before you get to the neutrino fog.”