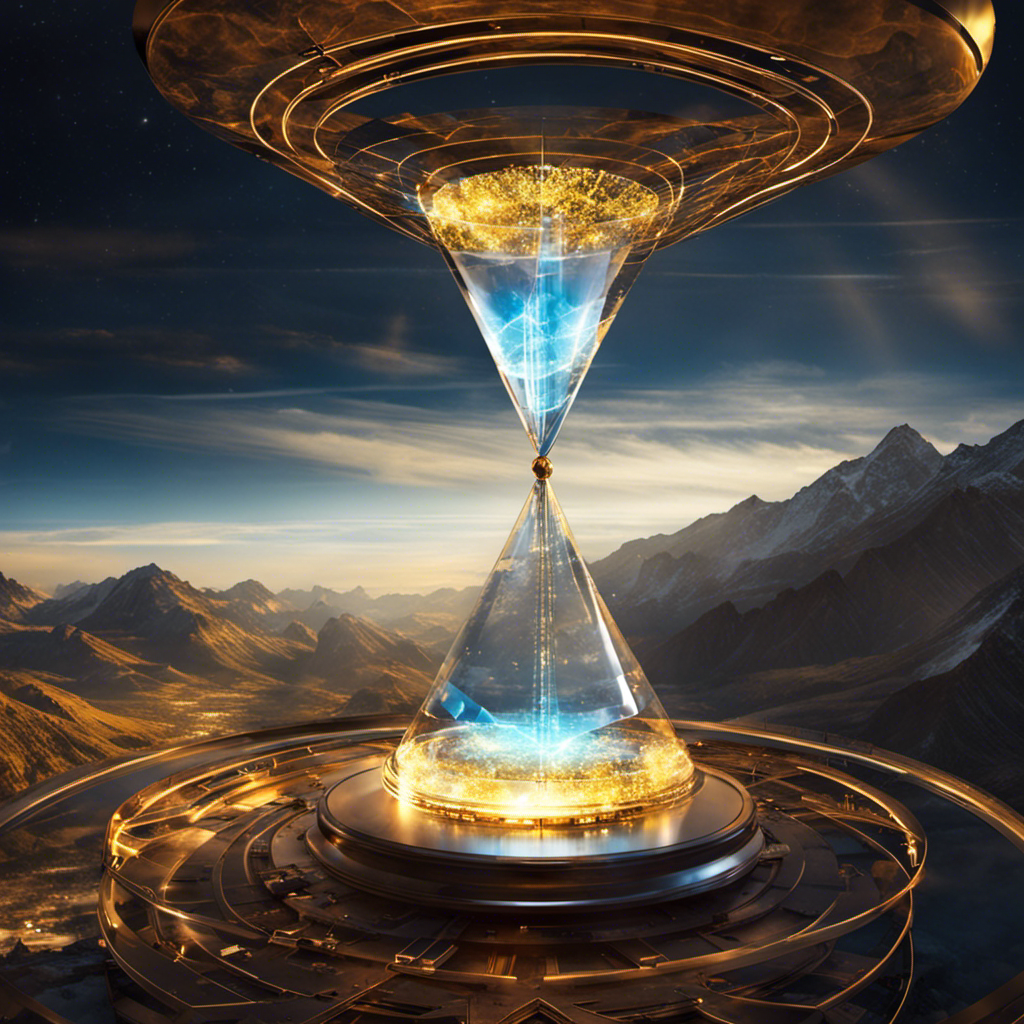
A new kind of time crystal has been made that could explain how these mysterious substances function and reveal the scale at which quantum effects kick in.
The existence of time crystals was proposed in 2012 by Frank Wilczek at the Massachusetts Institute of Technology. Normal crystals have patterns that repeat in three-dimensional space, creating a lattice structure. Wilczek imagined a crystal-like quantum system whose constituent parts would perpetually move to create patterns that repeat in time, instead. Because these theoretical substances required eternal movement without consuming energy, however, they appeared impossible under the laws of physics.
They didn’t remain theoretical for long, though. In 2017, two teams reported that they had found a way to make a kind of time crystal that oscillates, by repeatedly firing lasers at various substances. Although the resulting systems didn’t exactly follow Wilczek’s original definition, these “discrete” time crystals did have certain properties that oscillated, unrelated to the firing pattern of the laser.
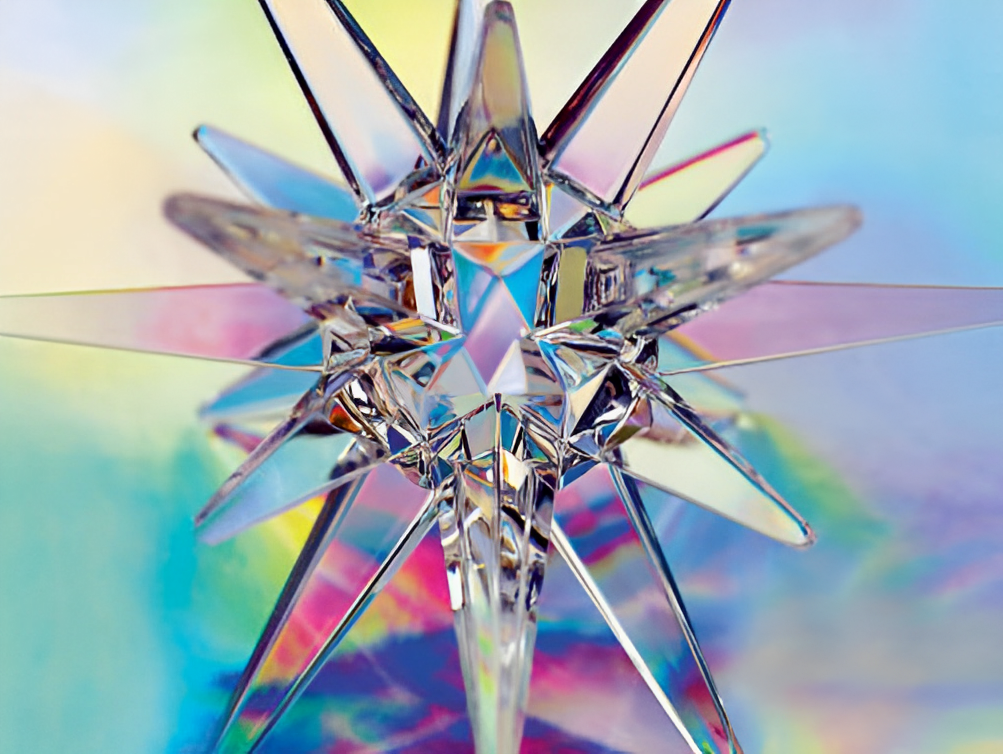
Now, Hans Keßler at the University of Hamburg in Germany and his colleagues have created a “continuous” time crystal using a constant source of laser light rather than the discrete bursts utilised in the earlier experiments. Keßler and his team cooled about 50,000 rubidium atoms to almost absolute zero and trapped them between two nanoscale mirrors that contained the laser beam.
The interactions between the atoms and the laser’s photons – particles of light – made both the distribution of the atoms and the intensity of the photons constantly oscillate.
This is similar to what happens in a more prosaic crystal such as ice, says Keßler. Liquid water looks the same in every direction, but when it freezes, a repeating lattice structure forms instead. And like with ice, where you can’t predict where the molecules will be located, you also can’t pinpoint when the repetition starts in the time crystal, due to a quirk of quantum mechanics.
“The analogy of our system to ice is that we know the frequency of the oscillation, but we cannot predict its time phase – when our time crystal gets formed. The observation… is closer to the original idea by Wilczek,” says Keßler.
Although other people have made continuous time crystals using “periodic drives” such as intermittent lasers, the continuity here is novel, says Samuli Autti at Lancaster University, UK. “One particularly impressive detail is that, because the driving technique used in the paper is not periodic, the authors can reliably draw a phase diagram of the time crystal, just like we can draw a diagram showing where water becomes solid as a function of pressure and temperature.”
Understanding such graphical representations of when the state of a substance changes means the system can be more reliably produced and experimented with.
This latest time crystal lasts only for about 10 milliseconds, but if that can be extended, then new quantum features could be introduced to the system as the number of atoms in it is varied. This could help us understand the scale at which quantum effects take hold, says Keßler.